Autonomic Control of Muscular Activity Before and After Exposure to Altitudes of 2000–3700 m
ISSN 0362-1197, Human Physiology, 2018, Vol. 44, No. 5, pp. 556–564. © Pleiades Publishing, Inc., 2018. Original Russian Text © R.S. Minvaleev, A.M. Sarana, S.G. Scherbak, A.S. Glotov, O.S. Glotov, O.P. Mamaeva, N.E. Pavlova, O.A. Guseva, A.I. Ivanov, A.I. Levitov, D.T. Summerfield, 2018, published in Fiziologiya Cheloveka, 2018, Vol. 44, No. 5, pp. 74–83.
Minvaleev R.S.a, Sarana A.M.a, Sсherbak S.G.a, Glotov A.S.a, Glotov O.S.a, Mamaeva O.P.b, Pavlova N.E.b, Guseva O.A.b, Ivanov A.I.a, Levitov A.I.c, Summerfield D.T.d
aSaint-Petersburg State University, Russia
bCity Hospital № 40, Saint-Petersburg, Russia
cEastern Virginia Medical School, USA
dHuman Integrative and Environmental Physiology Laboratory Mayo Clinic-Rochester, MN, USA
Abstract--- Every year more than 100 million people travel through mountains. Many of these travelers are middle aged and even elderly in whom there is minimal cardiovascular research. In this study we evaluated sympathico-vagal tone before and after exposure to middle altitudes.
13 subjects, 7 women and 6 men, ages 45 to 72 were evaluated before and after exposure to middle altitudes. Evaluations consisted of cardiac loading during echocardiography as well as calculating the vegetative tone by using the Kerdo index. Vegetative tone was analyzed using the Fisher’s maximum-likelihood estimation, after which the integral under the line of best fit was obtained with limits defined as the shortest loading test in seconds when compared to baseline.
Time to reach 85% maximally predicted cardiac rate increased for almost all subjects after exposure to middle altitudes. For the comparison of the vegetative control the integral of the fitting exponentials over time has been utilized. As demonstrated by an increase in the Kerdo index, 10 out of 13 of the subjects showed decrease followed by increase of the sympathetic responce as they acclimatized. Two of the three who did not demonstrate this shift has significant cardiovascular disorders which was discovered prior to exposure.
We have demonstrated decrease followed by increase of the sympathetic response during proper acclimatization. We demonstrated this with the vegetative Kerdo index in 10 healthy subjects. Furthermore the majority of the subjects with underlying cardiac pathology failed to demonstrate this shift.
Key words: graded stress test, altitude hypoxia, adaptation, vegetative Kerdo index
DOI: 10.1134/S0362119718030106
Introduction
Endurance training at middle altitudes is a common athletic practice which has been researched in a variety of studies [1-4]. Less researched however, is the short-term impact of these same altitudes on untrained middle-aged and elderly sojourners who are actively exerting themselves through trekking or hiking [5,6]. The paucity of data is surprising as the need for research in this area is clear given that year more than 100 million people travel through mountainous terrain [7]. Much of the current literature either focuses on the positive influence of hypoxic training on the cardiovascular system [8-10] or conversely it describes the cardiopulmonary risks and incidence of high altitude disease associated with short term or prolonged exposure to altitude [5-7, 11, 12].
One promising tool to fill the current knowledge gap is research on the autonomic nervous system controlled sympathico-vagal tone as determined by calculated heart rate variability [13, 14]. When loading tests are combined with direct visualization under stress echocardiography, real time assessment of autonomic control of exercise can be determined.
Unfortunately, researches on heart rate variability are mainly conducted at rest, due to the fact that the minimum recording length must be between 200 to 1000 or more cardiac cycles obtained in relatively stationary conditions (from 2-5 minutes to 24 hours) [15]. This does not allow for adequate estimations of a subject’s autonomic response in response dynamic conditions such as increasing physical exertion [16]. Even in case of ultrashort recording of the heart rate variability (less than one minute duration) one minute rest is usual [17,18] which does not allow real time registering of the vegetative response. Other methods of quantitative evaluation of autonomic nervous system also require a long-term recording of physiological characteristics [19, 20] and they cannot be used for an instant evaluation of autonomic control when exercising [21].
Invasive methods (such as microneurography) are also ill suited for the quantitative of the total autonomic control during the stress test. This is due to the fact that autonomic response in this case will not only reflect the whole body metabolism, but also local metabolic changes in the contracting muscle [22] Moreover stable position of the needle during exercise can not be guaranteed, affecting the accuracy of measurements[23].
Despite these limitations, in this study we have been able to compare autonomic control of exercise by performing stress echocardiographic loading tests both before and after exposure to the altitude of 2000 to 3700 m.
Subjects and methods
Subjects
13 low land research subjects (7 of them women) with ages ranging from 45 to 72 years old, all permanently residing at sea level, were recruited. Subjects included seven women. (see detailed description of the group in table 1). Over a two week time frame from April 26 to May 10 2016, all participants resided in the Himalayan middle mountains (Kullu valley, Himachal Pradesh state, India) at the altitude of 2000 to 3700 m. 3 more participants were subject to same conditions, including altitude from April 26 to May 10 of 2017. (Research expedition «Himalayas 2016» and «Himalayas 2017»).
This study was approved by the Institutional Review Board of Saint Petersburg State University (IRB00003875 – № 67, irb@spbu.ru). Written informed consent was translated into all relevant languages and cross translated to assure accuracy. Consent was signed by all participants after all questions had been answered by the scientific team.
Table 1. Descriptive characteristics of the test group (Name, Sex, Age, Weight, Height and Body mass index (BMI)
NN |
Name |
Sex |
Age (yr) |
Weight (kg) |
Height (cm) |
BMI |
1 |
М.Р. |
m |
50 |
82 |
182 |
24.8 |
2 |
М.Е. |
f |
51 |
73 |
164 |
27.1 |
3 |
S.G. |
m |
45 |
60 |
168 |
21.3 |
4 |
Е.G. |
m |
59 |
79 |
173 |
26.4 |
5 |
K.O. |
f |
49 |
70 |
160 |
27.3 |
6 |
V.D. |
m |
54 |
81 |
178 |
25.6 |
7 |
E.A. |
f |
48 |
54 |
162 |
20.6 |
8 |
K.I. |
f |
54 |
49 |
159 |
19.4 |
9 |
A.I. |
f |
52 |
85 |
165 |
31.2 |
10 |
B.G. |
f |
61 |
63 |
155 |
26.2 |
11 |
A.A |
m |
52 |
91 |
185 |
26.6 |
12 |
G.I. |
f |
51 |
68 |
172 |
23 |
13 |
B.Ju. |
m |
72 |
50 |
162 |
19 |
Mean ± SEM |
53.6±1.87 |
69.6±3.81 |
168±2.58 |
24.5±1 |
Daily excursions (trekking occurred for 10 consecutive days which all subjects participated in. These events lasted from 1-3 hours to 1-2 days and resulted in an increase in elevation, from base camp elevation of 2000 m to a maximum of 3700 m.
Protocol
Stress echocardiographic loading tests were conducted before and after the exposure to altitude. All subjects were examined at the same time points with the baseline pre-exposure test being carried out five days prior to ascent (at an altitude of sea level). All subjects were then re-examined on day three following return from altitude at the same location. Studies were performed using a horizontal bicycle ergometer (e-Bike EL&BP) with an expert class ultrasound system (Vivid E9 General Electric, ISA) on location at City Hospital №40 (Sestroretsk, Russia).
During each study participants performed a steady increase in physical exertion on a horizontal bicycle ergometer with a gradient of 25 W every 2 minutes, starting from 50 W, and continuing until a submaximal cardiac rate was reached. 85% of maximal cardiac rate was then calculated for each subject by the commonly accepted [24] formula (1):
Cardiac rate max = 220 – age (years) (1)
A continuous 12-lead ECG monitoring was obtained during the loading tests. In addition, at every incremental stage of increased work, systolic and diastolic blood pressure was recorded using auscultatory method by N. Korotkov.
Preliminary stress-echocardiographic examinations of the subjects revealed no signs of coronary ischemia as seen by regional wall motion abnormalities (stress test is negative for all subjects). Two subjects were found to have slight cardiac events during the testing. Subject E.A.(48) was found to have ventricular parasistolia, subject A.I.(52) had hypertensive reaction to the stressload as defined by preliminary stress-echocardiographic examination.
Vegetative Kerdo Index
Both systolic and diastolic blood pressures was recorded for each step of the loading tests, thus the vegetative Kerdo index [25] was calculated to evaluate changes in current autonomic tone by using the following formula (2):
V=1-D/R, (2)
where D is a diastolic pressure in mm Hg, R – heart rate (beats per minute), V – Kerdo index value. As a matter of convenience, we removed the uninformative coefficient of 100 from the formula, which only complicates the subsequent mathematical processing of the measured data.
Increased sympathetic tone is responsible for elevations in the ergotropic (energy-consuming) processes and increases of this tone can be defined as the energy debt required for increased autonomic control during exercise. It can be expressed through sympathico-vagal balance where the actual value of sympathetic tone in response to exertion can be found by calculating vegetative Kerdo index. At V < 0, the actual vegetative reactivity of the body is characterized as parasympathicotonia, at V > 0 – as sympathicotonia, at V = 0 – as vegetative balance [25].
In our previous work [26] we proved the applicability of Kerdo index in this form to measure current autonomic tone of sportsmen. In this study the vegetative Kerdo index was obtained at baseline prior to exposure to altitude and at day three after return.
Mathematical processing of the measured data
We compared the time required to achieve 85% of maximal cardiac rate and other echocardiographic parameters in response to loading tests before and after exposure to altitude by using Wilcoxon nonparametric paired test for linked samples meant for statistical processing of samples of a relatively law capacity (n = 13).
Due to measurements of autonomic control of exercise being carried out in real time, it was possible to find mathematical model (analytical form) fitting changes in time of vegetative tone in response to a standardized loading test. Fitting by a suitable exponential expression (3) with a subsequent quantitative estimation of autonomic control of exercise performed during standardized loading tests before and after exposure to middle altitudes was made by using Fisher’s maximum-likelihood estimation method.
V(t)=A1−A2∙e-kt , (3)
where A1, A2 and k the numerical coefficients, which are finding the method of maximum likelihood, t – time in seconds. To compare autonomic control of exercise before and after exposure to altitude we calculated the area ratio (integrals) under the obtained fitting exponents at an equal time span. Integration limits were defined by the shortest loading test (in seconds) of the baseline test performed prior to exposure to altitude.
Computation, statistical processing and graphical interpretations were made using mathematical programs Origin 8.6© and Derive 5.05©.
Results
The time to reach 85% of maximal cardiac rate during stress echocardiographic loading tests increased for all subjects with the reliability close to when post exposure times were compared to baseline rates (shown in table 2).
Table 2. Duration of increasing exertion (in seconds) before reaching submaximal (85% predicted) cardiac rate during stress echocardiographic loading tests before and after exposure to altitude.
NN |
Name |
Time, s |
|
Pre altitude exposure |
Post altitude exposure |
||
1 |
М.Р. |
552 |
751 |
2 |
М.Е. |
177 |
330 |
3 |
S.G. |
299 |
444 |
4 |
Е.G. |
259 |
454 |
5 |
K.O. |
282 |
389 |
6 |
V.D. |
412 |
700 |
7 |
E.A. |
312 |
459 |
8 |
K.I. |
183 |
330 |
9 |
A.I. |
169 |
223 |
10 |
B.G. |
224 |
325 |
11 |
A.A |
872 |
840 |
12 |
G.I. |
384 |
404 |
13 |
B.Ju. |
331 |
387 |
Median |
306 |
424 |
|
Type 1 error probability |
Р=0.000610352 |
*significance of differences (accurate P-value) was calculated by using Wilcoxon paired test for linked samples.
The following are the results of the mathematical processing of the autonomic control dynamics (vegetative Kerdo index) during a gradually increasing loading test. The mathematical processing is carried out with the use of exponential fitting.
Table 3. Integral estimation of autonomic control of exercise during a standardized loading test before and after exposure to middle altitudes for subject M.P (50), where DBP is a diastolic blood pressure, R – heart rate (beats per minute, bpm), A1, A2, k are coefficients of fitting exponent in the form V(t)=A1−A2∙e-kt, P is the significance of exponential model by FisherSnedecor criteria, S1 and S2 being integral ( surface under the curve) during the same time before and after the mountain altitude exposure.
1 |
2 |
3 |
4 |
5 |
6 |
7 |
8 |
|
Date |
Time (s) |
DBP (mm Hg) |
HR (bpm) |
Kerdo index |
Coefficients of fitting exponent in the form V(t)=A1−A2∙e-kt |
Integral estimation of autonomic control before (S1) and after (S2) exposure to middle altitudes |
S1/S2 |
|
21.04.2016 |
0 |
60 |
66 |
0.09 |
|
|
552 |
1.35 |
311 |
90 |
124 |
0.27 |
A1 |
0.27172 |
|||
352 |
90 |
123 |
0.27 |
A2 |
0.18062 |
|||
428 |
100 |
131 |
0.24 |
k |
0.00831 |
|||
475 |
100 |
134 |
0.25 |
Р |
0.000047 |
|||
505 |
100 |
141 |
0.29 |
|
|
|||
552 |
100 |
139 |
0.28 |
|
|
|||
|
|
|
|
|
|
|||
13.05.2016 |
1 |
80 |
62 |
-0.29 |
|
|
552 |
|
106 |
80 |
93 |
0.14 |
A1 |
0.24008 |
|||
224 |
80 |
99 |
0.19 |
A2 |
0.5362 |
|||
349 |
90 |
111 |
0.19 |
k |
0.01449 |
|||
473 |
90 |
118 |
0.24 |
Р |
0.0006 |
|||
652 |
100 |
133 |
0.25 |
|
|
|||
751 |
100 |
142 |
0.30 |
|
|
Measurements of arterial pressures and heart rate (beats per minute) were extracted at specific and consistent between studies (need to be more detailed here) time points every stress echocardiographic loading test report. Real-time point estimations of vegetative Kerdo index were then calculated (column 2-5 table 3). Exponential fitting of changes in time of vegetative Kerdo index’ numerical values was then performed by using the maximum-likelihood method (see column 6 table 3, where P is the significance of exponential model by FisherSnedecor criteria). Figure 1 is the graphical interpretation of the obtained fitting exponents of autonomic control of exercise during the performance of stress echocardiographic loading tests before and after exposure to altitude for subject M.P.
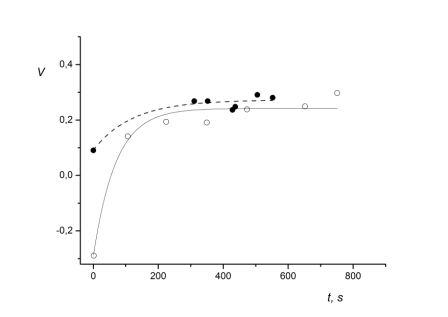
Fig. 1. Graphical interpretation of the autonomic control of exercise during the performance of loading tests before and after exposure to middle altitudes for subject M.P.
X-axis – time in seconds, Y-axis – vegetative Kerdo index;
● datum value of vegetative Kerdo index obtained during a loading test before exposure to middle altitudes;
- - - - fitting exponent in the form of before exposure to middle altitudes;
○ datum value of Kerdo index obtained during a loading test after exposure to middle altitudes;
─── fitting exponent in the form of after exposure to middle altitudes.
The following computation consisted of finding the ratio of areas determined by integrating the obtained exponential expressions with integration limits set from 0 to the duration of the loading test (552 seconds) performed five days prior to altitude exposure. The obtained area ratio – 1.35 – indicates that after exposure to the altitude the subject M.P.’s autonomic control of exercise shifted towards a decrease in sympathetic influence. This decrease corresponds to the physiological penalty of a loading test (ergotropic component). The decrease in sympathetic tone is illustrated in Fig. 1 where the exponent fitting point estimations of autonomic control during standardized loading tests before exposure to altitude is placed higher that the exponent fitting the results of autonomic control of the same loading test after exposure to altitude.
For the sake of completeness we had also included the data on the autonomic control before after ascend into the midrange altitude of the female participant G.I. (51) who had acquired the same route in May of 2017 ( see table 4).
Table 4. Integral estimation of autonomic control of exercise during loading tests before and after exposure to middle altitudes for subject G.I. (50). Abbreviations as in table 3.
1 |
2 |
3 |
4 |
5 |
6 |
7 |
6 |
|
Date |
Time (s) |
DBP (mm Hg) |
HR (bpm) |
Kerdo index |
Coefficients of fitting exponent in the form V(t)=A1−A2∙e-kt |
Integral estimation of autonomic control before (S1) and after (S2) exposure to middle altitudes |
S1/S2 |
|
20.04.2017 |
0 |
60 |
57 |
-0.05 |
|
|
384 |
≈1.6 |
85 |
60 |
94 |
0.36 |
|
|
|||
187 |
70 |
112 |
0.38 |
A1 |
0.46306 |
|||
271 |
70 |
125 |
0.44 |
A2 |
0.51195 |
|||
302 |
70 |
129 |
0.46 |
k |
0.01624 |
|||
384 |
70 |
146 |
0.52 |
Р |
0.00155 |
|||
|
|
|
|
|
|
|||
11.05.2017 |
0 |
80 |
55 |
-0.45 |
|
|
384 |
|
87 |
80 |
97 |
0.18 |
|
|
|||
189 |
80 |
110 |
0.27 |
A1 |
0.39181 |
|||
270 |
80 |
120 |
0.33 |
A2 |
0.84068 |
|||
332 |
80 |
134 |
0.40 |
k |
0.01388 |
|||
404 |
80 |
142 |
0.44 |
Р |
0.00219 |
|||
|
|
|
|
|
|
Fig. 2 is the graphical interpretation of the obtained fitting exponents of autonomic control of exercise during the performance of stress echocardiographic loading tests before and after exposure to altitude for subject G.I.
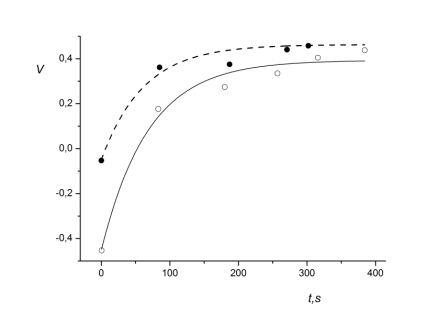
Fig. 2. Graphical interpretation of autonomic control of exercise during loading tests before and after exposure to middle altitudes for subject A.I. (52). Symbols as in Fig.1
Similar computations were carried out for the other 11 subjects. Two out of three subjects who did not have a decrease of ergotropic component in autonomic control of exercise had serious cardiovascular disorders discovered during the preliminary all-around examination (it was found that subject E.A. (48) has ventricular parasistolia and subject A.I. (52) has hypertonia). To illustrate failed adaptation, we present the results of integral estimation of autonomic control of exercise during loading tests for subject A.I. (52): table 5 and corresponding Figure 3.
Table 5. Integral estimation of autonomic control of exercise during loading tests before and after exposure to middle altitudes for subject A.I. (52).
1 |
2 |
3 |
4 |
5 |
6 |
7 |
8 |
|
Date |
Time (s) |
DBP (mm Hg) |
HR (bpm) |
Kerdo index |
Coefficients of fitting exponent in the form V(t)=A1−A2∙e-kt |
Integral estimation of autonomic control before (S1) and after (S2) exposure to middle altitudes |
S1/S2 |
|
21.04.2016 |
1 |
85 |
96 |
0.11 |
|
|
312 |
≈0.8 |
7 |
85 |
97 |
0.12 |
A1 |
0.21586 |
|||
68 |
90 |
116 |
0.22 |
A2 |
0.1126 |
|||
128 |
100 |
125 |
0.20 |
k |
0.04391 |
|||
174 |
100 |
131 |
0.24 |
Р |
0.0000045 |
|||
223 |
110 |
137 |
0.20 |
|
|
|||
301 |
110 |
139 |
0.21 |
|
|
|||
312 |
110 |
142 |
0.23 |
|
|
|||
|
|
|
|
|
|
|||
13.05.2016 |
1 |
80 |
86 |
0.07 |
|
|
312 |
|
67 |
80 |
107 |
0.25 |
A1 |
0.27674 |
|||
189 |
85 |
120 |
0.29 |
A2 |
0.21381 |
|||
221 |
90 |
120 |
0.25 |
k |
0.03248 |
|||
310 |
100 |
130 |
0.23 |
Р |
0.000065 |
|||
352 |
100 |
133 |
0.25 |
|
|
|||
457 |
100 |
146 |
0.32 |
|
|
|||
459 |
100 |
148 |
0.32 |
|
|
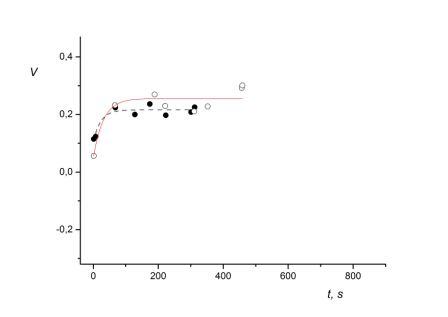
Fig. 3 Graphical interpretation of autonomic control of exercise during loading tests before and after exposure to middle altitudes for subject A.I. (52). Symbols as in Fig.1
The ratio of autonomic control’s integral estimations for subject A.I. (52) equals 0.8. This denotes the increase in sympathetic tone in response to a standardized loading test after exposure to altitude. Thus, despite the fact that the time required to reach submaximal cardiac rate in response to standardized loading tests has increased for all participants exposed to middle altitudes, the autonomic control has showcased opposite trends in healthy subjects and those who have cardiovascular disorders. Results of the integral estimation of exponential fitting of autonomic control during loading tests showcase that after exposure to altitudes of 2000-3700 m, adaptation (as measured by adequate autonomic control of exercise) to the stressor of altitude occurred for only for 10 out of 13 subjects. In these subjects the area under the corresponding fitting exponent decreased after exposure to the altitude of 2000-3700 m (see table 6).
Table 6. The ratio of integral estimation of autonomic control before (S1) and after (S2) exposure to middle altitudes for all 13 subjects (in the last three subjects the ratio of areas less than or equal to 1).
NN |
Name |
S1/S2 |
1 |
М.Р. |
1.35 |
2 |
М.Е. |
1.5 |
3 |
S.G. |
1.08 |
4 |
Е.G. |
1.4 |
5 |
K.O. |
1.23 |
6 |
V.D. |
1.47 |
7 |
E.A. |
1.3 |
8 |
K.I. |
1.22 |
9 |
A.I. |
1.6 |
10 |
B.G. |
1.15 |
11 |
A.A |
1 |
12 |
G.I. |
0.8 |
13 |
B.Ju. |
0.94 |
Discussion
It is known that acute exposure to altitude raises sympathetic tone of the autonomic nervous system [13,14]. This is manifested by a corresponding increase in arterial pressures [12,27,28] as well as increased levels of catecholamine in blood [29-34]. These in its turn raise the risk of ventricular arrhythmia and oxymortia [6,7,11], especially for those with previous myocardial infarctions [35]. Pathological consequences of chemo- and baroreflex sympathico-adrenal activation when climbing to altitude [36] allow us to suggest that opposite mechanisms can be used to adequately regulate autonomic control during acute adaptation to altitude hypoxia [37].
Decreases in cardiac rate [38,39] observed when rising are directly linked to an increase in parasympathetic effect on heart. This has been shown in studies which have implemented blockade of muscarinic cholinoreceptors both at rest and during physical activity before and after exposure to an altitude in which it was shown that the reduction in exercise HR after acclimatization to high altitude in healthy, active individuals is due to enhanced parasympathetic neural tone [40]. In a number of studies, spectral analysis of heart rate variability found that prolonged adaptation to an altitude in healthy subjects results in a compensatory increase in vagal (parasympathetic) effect on the heart following an initial increase in sympathetic tone. The transition from a predominant parasympathetic to a sympathetic state can be viewed as one of the signs of a successful adaptation to the altitude [41,42, 43]. However recently the leading role of the sympathetic nervous system in adaptation to altitude has been subject to reasonable doubt [44, 45].
In a 2001 Japanese study, Kanai et al. [46], conducted a similar investigation to ours, using the same altitudes (at 2700 and 3700 m above sea level) and on tourists in the same age range.
They showed an already known dominance of sympathetic over parasympathetic activity when rising from a supine to standing position by using a spectral analysis of heart rate variability. However, the researchers themselves admitted that their data (or conclusions) was limited due to the need to account for the influence of operating stress since additional physical exertion can cause further change in the autonomic nervous system activity. We attempted to account for this need by using stress echocardiography loading tests before and after exposure to altitudes.
In the current study we have shown a shift of autonomic control of exercise towards decreased sympathetic tone in response to a gradually increasing physical exertion. This occurred in the majority of subjects after 10-days of exposure to altitudes of 2000-3700 m, signifying a reduction in the risk of cardiovascular disorders and an increasing involvement of parasympathetic system as an autonomous antagonist to the sympathico-adrenal system. Revealing the consistent pattern of autonomic control in response to physical exertion was made possible because of the new method of integral evaluation of autonomic control of exercise using vegetative Kerdo index [25]. Linking point measurements to point times during loading tests allowed us to perform an adequate exponential fitting and then comparing obtained analytical expressions of autonomic control both before and after exposure to the altitude.
In a 2010 study similar to ours, Danish researchers studied heart reactions to physical exertion after a short-term exposure to the altitude of 4200 m above sea level. Their investigation revealed no differences in heart reactions of people who had suffered from myocardial infarction in comparison with healthy controls [47]. In contrast with the current study we found significant differences in sympathetic nervous system reaction in response to physical exertion between relatively healthy subjects and those who had current cardiovascular disorders in their case history. More specifically, two subjects (with arrhythmia and hypertonia correspondingly) did not demonstrate the decrease in the tone of the sympathetic nervous system in response to physical exertion after exposure to the altitude, although it requires additional studies on a larger number of subjects.
Conclusions
- Quantitative estimation of autonomic control of exercise during physical activity can be carried out by using vegetative Kerdo index.
- Adaptation to a week-long exposure to the altitude of 2000 to 3700 m lowers the level of sympathetic nervous system activity in autonomic control of exercise in response to standardized exertion for healthy people and do not lower it for people with cardiovascular disorders.
Funding: This study was funded by the Russian Science Foundation [No14-50-00069] and Saint-Petersburg State University.
Conflicts of interest: The authors declare that they have no conflict of interest.
Acknowledgments
We thank Arkhipova IV, the general director and the CEO of the Faraon studio of historical films and the organizer of international research expeditions to the Himalayas, carried out through the project ‘‘V poiskakh utrachennykh znanii’’ (In Search for Lost Knowledge).
References
- Rusko, H.K., Tikkanen, H.O., Peltonen, J.E. Altitude and endurance training // J. Sports Sci. 2004, vol.22, № 10, p. 928.
- Friedmann-Bette, B. Classical altitude training // Scand. J. Med. Sci. Sports. 2008, vol. 18, Suppl. 1, p.11.
- Saunders, P.U., Pyne, D.B., Gore, C.J. Endurance training at altitude // High Alt. Med. Biol. 2009, vol.10, № 2, p.135.
- Constantini, K., Wilhite, D.P., Chapman, R.F. A Clinician Guide to Altitude Training for Optimal Endurance Exercise Performance at Sea Level // High Alt. Med. Biol. 2017, vol.18, № 2, p.93.
- Veglio, M., Maule, S., Cametti, G., Cogo, A., et al. The effects of exposure to moderate altitude on cardiovascular autonomic function in normal subjects // Clin. Auton. Res. 1999, vol.9, № 3, p.123.
- Riley, C.J., Gavin, M. Physiological Changes to the Cardiovascular System at High Altitude and Its Effects on Cardiovascular Disease // High Alt. Med. Biol. 2017, vol.18, №2. P.102.
- Higgins, J.P, Tuttle, T, Higgins, JA. Altitude and the heart: is going high safe for your cardiac patient? // Am. Heart J. 2010, vol. 159, № 1, p.25.
- Minvaleev, R.S.. [A comparison of rate human lipid profile changes at moderate altitude. Article in Russian] // Fiziol. Cheloveka. 2011, vol.37, №3, p.103.
- Wee, J., Climstein, M. Hypoxic training: Clinical benefits on cardiometabolic risk factors // J. Sci. Med. Sport. 2015, vol.18, № 1, p.56.
- Gutwenger, I., Hofer, G., Gutwenger, A.K, Sandri M., et al. Pilot study on the effects of a 2-week hiking vacation at moderate versus low altitude on plasma parameters of carbohydrate and lipid metabolism in patients with metabolic syndrome // BMC Res. Notes. 2015, vol.28, № 8, p.103.
- Seccombe, L.M., Peters, M.J. Physiology in medicine: acute altitude exposure in patients with pulmonary and cardiovascular disease // J. Appl. Physiol. (1985). 2014, vol.116, № 5, p.478.
- Parati, G., Ochoa, J.E., Torlasco, C., Salvi, P., et al. Aging, High Altitude, and Blood Pressure: A Complex Relationship // High Alt. Med. Biol. 2015, vol.16, № 2, p.97.
- Perini, R., Milesi, S., Biancardi, L., Veicsteinas, A. Effects of high altitude acclimatization on heart rate variability in resting humans // Eur. J. Appl. Physiol. Occup. Physiol. 1996, vol.73, № 6, p. 521.
- Bernardi, L., Passino, C., Spadacini, G., Calciati, A., et al. Cardiovascular autonomic modulation and activity of carotid baroreceptors at altitude // Clin. Sci. (Lond). 1998, vol. 95, № 5, p.565.
- Heart rate variability: standards of measurement, physiological interpretation and clinical use. Task Force of the European Society of Cardiology and the North American Society of Pacing and Electrophysiology // Circulation. 1996, vol. 93, № 5, p.1043.
- Casadei, B., Cochrane, S., Johnston, J., Conway, J., et al. Pitfalls in the interpretation of spectral analysis of the heart rate variability during exercise in humans // Acta Physiol. Scand. 1995, vol.153, №2, p.125.
- Nussinovitch U., Elishkevitz K.P., Katz K., Nussinovitch M., et al. Reliability of ultra-short ECG indices for heart rate variability. Annals of Noninvasive Electrocardiology. 2011. V.16. № 2. P.117.
- Nakamura F.Y., Flatt A.A., Pereira L.A., Ramirez-Campillo R., et al. Ultra‑Short‑Term Heart Rate Variability is Sensitive to Training Effects in Team Sports Players. J Sports Sci Med. 2015. V.14. № 3. P. 602.
- Jaradeh S.S., Prieto T.E. Evaluation of the autonomic nervous system. Phys Med Rehabil Clin N Am. 2003. V.14. № 2. P.287.
- Low P.A. Laboratory evaluation of autonomic function. Suppl Clin Neurophysiol. 2004. V.57. P.358.
- Novak P. Quantitative autonomic testing. J Vis Exp. 2011. V.53. P.2502.
- Saito M., Mano T. Exercise mode affects muscle sympathetic nerve responsiveness. Jpn J Physiol. 1991. V.41. №1. P.143.
- Ichinose M., Saito M., Fujii N., Ogawa T., et al. Modulation of the control of muscle sympathetic nerve activity during incremental leg cycling. J Physiol. 2008. V.586. № 11. P.2753.
- Tavrovskaya Т.V. [Bicycle ergometry. Book in Russian] St. Petersburg: Department of Faculty Therapy of Altai State Medical University. 2007. P.20
- Kérdö I. Ein aus Daten der Blutzirkulation kalkulierter Index zur Beurteilung der vegetativen Tonuslage. Acta Neuroveg (Wien). 1966. V.29. № 2. P.250.
- Namozova S.Sh., Khubbiev Sh.Z., Minvaleev R.S., Shadrin L.V.. [Monitoring of functional state of national teams’ members in university sport pedagogical management system: selection of relevant criteria. Article in Russian]. Theory and Practice of Physical Culture. 2016. №4. P.20.
- Wolfel E.E., Selland M.A., Mazzeo R.S., Reeves J.T. Systemic hypertension at 4,300 m is related to sympathoadrenal activity. J Appl Physiol (1985). 1994. V.76. № 4. P.1643.
- Mingji C., Onakpoya I.J., Perera R., Ward A.M., et al. Relationship between altitude and the prevalence of hypertension in Tibet: a systematic review. Heart. 2015. V.101. № 13. P.1054.
- Cunningham W.L, Becker EJ, Kreuzer F. Catecholamines in plasma and urine at high altitude. J Appl Physiol. 1965. V.20. № 4. P.607.
- Mazzeo R.S., Bender P.R., Brooks G.A., Butterfield G.E., et al. Arterial catecholamine responses during exercise with acute and chronic high-altitude exposure. Am J Physiol. 1991. V.261. № 4. Pt 1. P.E419.
- Mazzeo R.S., Wolfel E.E., Butterfield G.E., Reeves J.T. Sympathetic response during 21 days at high altitude (4,300 m) as determined by urinary and arterial catecholamines. Metabolism. 1994. V.43. № 10. P.1226.
- Antezana A.M., Kacimi R., Le Trong J.L., Marchal M., et al. Adrenergic status of humans during prolonged exposure to the altitude of 6,542 m. J Appl Physiol (1985). 1994. V.76. № 3. P.1055.
- Rostrup M. Catecholamines, hypoxia and high altitude. Acta Physiol Scand. 1998. V.162. № 3. P.389.
- Mazzeo R.S., Reeves J.T. Adrenergic contribution during acclimatization to high altitude: perspectives from Pikes Peak. Exerc Sport Sci Rev. 2003. V.31. № 1. P.13.
- Messerli-Burgy N., Meyer K., Steptoe A., Laederach-Hofmann K. Autonomic and cardiovascular effects of acute high altitude exposure after myocardial infarction and in normal volunteers. Circ J. 2009. V.73. № 8. P.1485.
- Hansen J., Sander M. Sympathetic neural overactivity in healthy humans after prolonged exposure to hypobaric hypoxia. J Physiol. 2003. V.546 (Pt 3). P.921.
- Sevre K., Bendz B., Hankø E., Hauge A., et al. Reduced autonomic activity during stepwise exposure to high altitude. Acta Physiol Scand. 2001. V.173. № 4. P.409.
- Åstrand P.O., Åstrand I. Heart rate during muscular work in man exposed to prolonged hypoxia. J Appl Physiol. 1958. V.13. №1. P.75.
- Hartley L.H., Vogel J.A., Cruz J.C. Reduction of maximal exercise heart rate at altitude and its reversal with atropine. J Appl Physiol. 1974. V.36. №3. P.362.
- Boushel R., Calbet J.A., Rådegran G., Sondergaard H., et al. Parasympathetic neural activity accounts for the lowering of exercise heart rate at high altitude. Circulation. 2001. V.104. P.785.
- Hughson R.L., Yamamoto Y., McCullough R.E., Sutton J.R., et al. Sympathetic and parasympathetic indicators of heart rate control at altitude studied by spectral analysis. J Appl Physiol (1985). 1994. V.77. № 6. P.2537.
- Bhaumik G., Dass D., Bhattacharyya D., .et al. Heart rate variabilty changes during first week of acclimatization to 3500 m altitude in Indian military personnel. Indian J Physiol Pharmacol. 2013. V.57. № 1. P.16.
- Levine B.D., Zuckerman J.H., deFilippi C.R. Effect of high-altitude exposure in the elderly: the Tenth Mountain Division study. Circulation. 1997. V.96. №4. P.1224
- Sander M. Does the Sympathetic Nervous System Adapt to Chronic Altitude Exposure? Adv Exp Med Biol. 2016. V.903. P.375.
- Siebenmann C., Rasmussen P., Hug M., Keiser S., et al. Parasympathetic withdrawal increases heart rate after 2 weeks at 3454 m altitude. J Physiol. 2017. V.595. № 5. P.1619.
- Kanai M., Nishihara F., Shiga T., Shimada H., et al. Alterations in autonomic nervous control of heart rate among tourists at 2700 and 3700 m above sea level. Wilderness Environ Med. 2001. V.12. № 1. P.8.
- de Vries S.T., Komdeur P., Aalbersberg S., et al. Effects of altitude on exercise level and heart rate in patients with coronary artery disease and healthy controls. Neth Heart J. 2010. V.18. № 3. P.118.
Файлы для скачивания: